Plasma sources
Plasma acceleration requires reliable and well known plasma sources. The plasma properties of any plasma-based acceleration experiment have to match the requirements of the experimental conditions. The first problem to overcome is the ionization of the neutral atoms to produce plasma. While for the laser-driven acceleration the laser is usually intense enough to ionize the gas until a degree at which the ionization is no more a problem for pump depletion, for bunch-driven PWFA this is not necessarily true and the plasma source must to take into account this problem.
One of the main requirements to a prepared plasma structure is fine external tuning of the plasma parameters. These parameters are very important for injection and propagation of the laser/electron beams in the plasma channel and in the case of laser wake field acceleration, matching of the accelerated electrons phase to the wake-field. Those parameters include: radial and longitudinal plasma density distribution, plasma composition and degree of ionization, the plasma channel spatial dimensions and temporal dynamics, the plasma temperature. These parameters are very important for injection and propagation of the laser/electron beams in the plasma channel, in particular for matching the accelerated electrons phase to the wake-field along the entire acceleration path, which may require several centimeters of plasma. Multistage capillary discharge scheme has made possible easy online control of the most of these parameters, allowing to fit all the plasma characteristics to the different stages of the accelerator.
In external injection schemes, both laser or beam driven, a uniform, “low” (1016 – 1017 cm-3) plasma density for centimeter scale length is required, with sufficient transverse uniformity and relatively low temperature (<10 eV). On the other hand, self-injection, schemes require higher density (1019 cm-3), but still with long and uniform distribution.
In order to control the plasma acceleration process and the particle bunch properties, very reliable plasma sources are mandatory.
Capillary
Our main efforts are devoted to the research on hydrogen filled capillaries as plasma source.
The main characteristics of the gas filled capillary sources is to provide a pre-ionized plasma channel for plasma-based acceleration experiments. In these devices a current pulse of several amperes passes through the capillary filled with gas at pressure of few tens of millibar. The current ionizes the gas, preforming the plasma channel before the interaction with the driver beams.
The necessity to use capillaries rises from the need to extend the interaction length of the drivers with the plasma. Capillaries indeed allow for longer and almost constant density profile up to centimeter scale. This kind of capillaries has been primarily used for LWFA because of the typical parabolic transverse density profile which allows to guide the laser radiation for distances longer than the Rayleigh length. Nevertheless, the high ionization level which can be reached by the discharge let to reduce the ionization losses acting on the driver(s), and it is particularly suitable for low energy particle drivers which are not intense enough to ionize the gas.
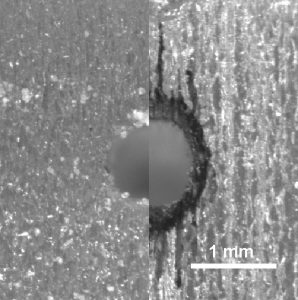
Entrance of the capillary after the print (left) and after more than 55 thousand shots (right). The burnt on the entrance is probably caused by the electrons impinging on the plastic during the alignment.
These capillaries are usually made of hard materials, like alumina, quartz, diamond or sapphire but we have also investigated the possibility to produce 3D printed plastic capillaries [4] which have survived for more than 200 thousand shots and the effects of the ablation on the plasma density distribution is not evident and the capillary can still be used. The use of these capillaries will significantly reduce the cost and time for prototyping, allowing to easily manipulate their geometry, laying another building block for future cheap and compact particle accelerators.
Compared with other plasma sources, gas filled capillaries allow to control the properties of the plasma channel locally by varying the capillary shape or by timing the discharge with the beams to wait for the desired plasma profile during the interaction. This let to have homogeneous plasma profile even at low densities of the order of 1016-1017 cm-3 as requested by our external injection experiments. However, electron density creation inside these capillaries is sensitive to many parameters, such as the walls composition, the shape of the electrodes or the resistances of the discharge circuit, which require fine measurements and are not easy to control during the device manufacturing. The definitive characterization of gas-filled capillary discharge must be always performed experimentally and with our measurements setup (described below) allows to measure its temporal as well as spatial evolution.
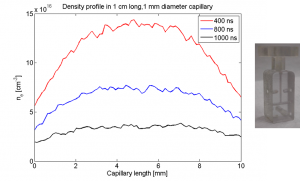
Temporal and spatial characterization of the longitudinal plasma density along an hydrogen based plasma confined into a 10 mm long capillary [1].
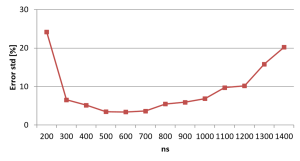
Variation on values averaged over 14 mm in the middle of the capillary for different time delay with respect to the discharge trigger. The variation has been calculated by analyzing 5 images per each shot [1].
Plasma diagnostics
Any plasma source requires different diagnostic tools to determine the principal plasma characteristics needed for the acceleration. On-line and non-perturbing methods suitable for small ($\sim$1 mm thick or less) plasmas are mandatory. Due to the small dimension of the plasma, mechanical probing is unpractical since they interfere with the plasma changing its local conditions. On the opposite, spectroscopic and interferometric techniques fit very well these requirements.
Single shot and spatially resolved measurements are crucial for the optimization of the beam quality during the acceleration.
Plasma spectroscopy
Plasma spectroscopy
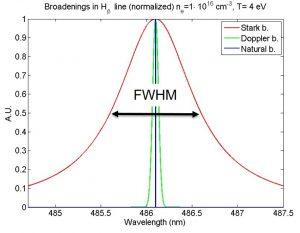
Comparison between the Stark, Doppler and Natural broadening (caused by the uncertainty principle) of the Balmer beta line in a hydrogen plasma of density 1016 cm-3 and temperature of 4 eV.
Light emitted by plasma allows to reconstruct the electron density from spectroscopy on emission line broadening due to Stark-Lo Surdo effect. Ionized hydrogen emits in visible range four lines of the so-called Balmer series. The broaden of those lines depends on many mechanisms:
- Doppler broadening caused by thermal particle motion, depends on plasma temperature.
- Stark broadening caused by the emitter interaction with the electric field produced by nearby charges.
- Other broadenings that for our plasmas, in the visible light at temperatures of the order of 1-4 eV can be neglected.
In general, the emitted radiation properties depend on the plasma characteristics, and its analysis allows to reconstruct the plasma conditions in the vicinity of the emitters.
Hydrogen plasma (and even hydrogen doped plasma) produces strong lines in the visible range, whose properties can be studied with good reliability with spectroscopic measurements and the light emitted do not suffer from capillary diffraction. The spectral analysis of these lines allows to reconstruct the electron density of the surrounding plasma due to the Stark effect. This technique can be used also in case of ablative capillaries, where multi-species of plasma are produced (including hydrogen), allowing the retrieval of plasma properties around the emitter.
With the system we have implemented we can detect the spatial and temporal evolution of the plasma online in single shot analysis inside and even outside the capillary itself allowing to study the interaction of the electron beam with the entire plasma profile.
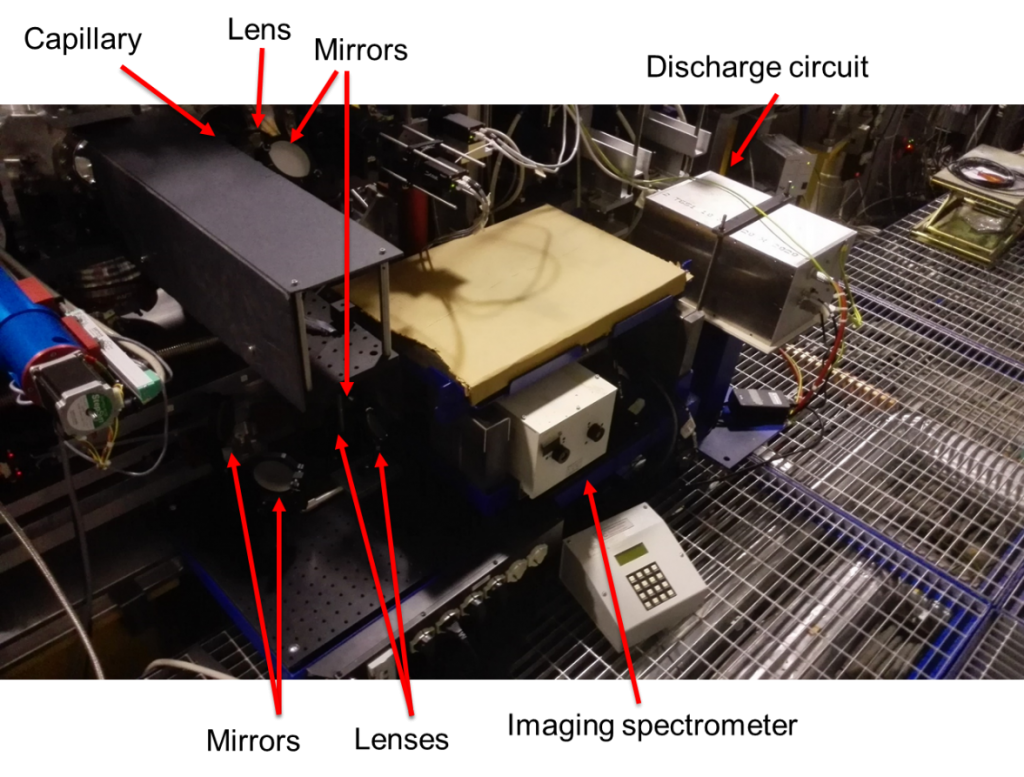
Stark broadening measuring system implemented in the SPARC_LAB bunker for the online measurement of the plasma density during the acceleration experiments.
Minimum spatial resolution | 0.01 – 0.1 mm |
Minimum temporal resolution | 10 – 100 ns |
Density range | 1015 – 1019 cm-3 |
Plasma interferometry
Plasma interferometry
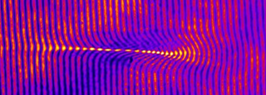
Example of an interferogram.
Interferometric methods use the dependence of the refractive index on the density in a transparent medium. The refractive index variation can be detected by experimentally measuring the dephasing of a probe beam caused by the different phase velocity of the light propagating into the plasma. The phase velocity $v_{\phi}$ indeed is inversely related to the refractive index $\eta$ ($v_{\phi}=c/\eta$) and by analyzing its variation it is possible to recover the plasma density crossed by the beam. To properly reconstruct the density the probe laser should not undergo to more complicated effects which are hard to discern by the signal. These effects can be the change of polarization produced by plasma anisotropy or refraction caused for example by the boundary walls of the plasma. Moreover, the signal should be intense enough to produce a significant shift of the fringes $\Delta \phi$, given by the formula
$\Delta \phi \simeq \frac{2\pi}{\lambda}\int_{0}^{L}\frac{n\left(z\right)}{n_c} \partial z$
where $\lambda$ is the laser wavelength, $L$ the plasma dimension, $n_e$ the local plasma density (function of the position $z$) and $n_c$ the critical plasma density, defined as $n_c=\left(m_e \omega_0^2 \right)/\left(4\pi e^2 \right)$. A typical setup used for the interferometric measurement based on the Mach-Zehnder interferometer.
Interferometric technique can be considered as a complementary technique respect to the Stark broadening analysis, and both can be implemented for online single shot measurements on the same capillary. Moreover, it allows for higher temporal resolution (of the femtoseconds range, depending on the probe pulse length) and can be implemented for the control of the plasma density during the beam-plasma interaction.
Plasma profile outside the capillary
Plasma profile outside the capillary
By measuring the typical parameters of the shock regime created at the ends of the capillary, as the Mach number and plasma supersonic expansion velocity, it is possible to estimate temperature and pressure both inside and outside the capillary (where will be created the so-called plasma plumes).
The movie shows the plasma expansions from the orifices of the capillary into the vacuum during the gas ionization.
Dielectric wake fields
Plasma wake-field acceleration experiments are performed at the SPARC_LAB test facility by using a gas-filled capillary plasma source composed of a dielectric material. Electrons can reach GeV-level energy in a few centimeters, with an accelerating gradient of several orders of magnitude larger than that provided by conventional techniques. In this acceleration scheme, wake fields produced by passing electron beams through dielectric structures can determine a strong beam instability that represents an important hurdle towards the capability to focus high-current electron beams in the transverse plane. For these reasons, the estimation of the transverse wake field amplitudes assumes a fundamental role in the implementation of the particle wake-field acceleration. In the following description, it is possible to observe the effects produced by dielectric wake fields when the electron beam goes through the dielectric capillary used at SPARC_LAB to implement the plasma acceleration. The experimental set-up uses cylindrical hydrogen-discharge plasma capillaries. The plasma channel achieved inside the capillary is 30 mm in length and 0.5 mm in radius. Two gas inlets of 0.3 mm in radius feed the plasma channel with hydrogen gas.
With regard to the implementation of the plasma-based accelerators by using gas-filled capillary plasma sources, we are interested in the estimation of the transverse wake fields created inside a dielectric capillary by a single bunch in order to determine how such electric fields affect the stability of the bunch itself. Therefore, our study concerns the analysis of the wake fields acting on a single bunch, which are created by the bunch’s head and act on the bunch itself, especially to the tail, as it is possible to observe in the short movie below. This movie is referred to a bunch of length 270 µm, by using a dielectric capillary of 30 mm in length and 0.5 mm in radius.
This phenomenon depends on different parameters, related to both the geometric properties of the capillary, as the radius of the plasma channel, and the beam properties, in particular to the bunch length. Since the wake field magnitude is inversely proportional to the bunch length and, in order to perform the plasma acceleration, it is necessary to produce very short bunches, such parameter strongly affects the wake field excitation inside a dielectric capillary, as shown in the figure below, that represent the simulation of the transverse wake field at different bunch lengths.
Publication highlights:
- “Spectroscopic measurements of plasma emission light for plasma-based acceleration experiments.”, F. Filippi et al., Journal of Instrumentation vol. 11 (2016) pag. C09015, doi: 10.1088/1748-0221/11/09/C09015.
- “Electron density measurement in gas discharge plasmas by optical and acoustic methods”, A. Biagioni et al., Journal of Instrumentation vol. 11 (2016) pag. C08003, doi: 10.1088/1748-0221/11/08/C08003
- “Betatron radiation based diagnostics for plasma wakefield accelerated electron beams at the SPARC_LAB test facility”, V. Shpakov et al., Nucl. Instrum. and Meth. in Phys. Res. Section A vol. 829 (2016) pag. 330, doi: 10.1016/j.nima.2016.02.074
- “Plasma density characterization at SPARC_LAB through Stark broadening of Hydrogen spectral lines”, F. Filippi et al., Nucl. Instrum. and Meth. in Phys. Res. Section A vol. 829 (2016) pag. 326, doi: 10.1016/j.nima.2016.02.071
- “Gas-filled capillaries for plasma-based accelerators”, F. Filippi et al., J. Phys.: Conf. Series vol. 874 (2017) pag. 012036, doi: 10.1088/1742-6596/874/1/012036
- “Plasma ramps caused by outflow in gas-filled capillaries.”, F. Filippi et al., Nucl. Instrum. and Meth. in Phys. Res. Section A vol. 909 (2018) pag. 346, 10.1016/j.nima.2018.02.102
- “Tapering of plasma density ramp profiles for adiabatic lens experiments”, F. Filippi et al., Nucl. Instrum. and Meth. in Phys. Res. Section A vol. 909 (2018) pag. 339, 10.1016/j.nima.2018.04.037
- “Wake fields effects in dielectric capillary”, A. Biagioni et al., Nucl. Instrum. and Meth. in Phys. Res. Section A vol. 909 (2018) pag. 247, doi: 10.1016/j.nima.2018.01.028
- “3D-printed capillary for hydrogen filled discharge for plasma based experiments in RF-based electron linac accelerator”, F. Filippi et al., Rev. Sci. Instrum. vol. 89 (2018) pag. 083502, doi:10.1063/1.5010264
- “High-voltage pulser to produce plasmas inside gas-filled discharge capillaries”, A. Biagioni et al., SPARC_LAB internal note (2019), SPARC-PL-19/001
- “Vacuum system design for plasma wakefield acceleration at SPARC_LAB test facility”, A. Biagioni et al., SPARC_LAB internal note (2021), PL-21/001
- “Beam-based characterization of plasma density in a capillary-discharge waveguide”, S. Romeo et al., AIP Advances, vol. 11 (2021) pag. 065217, doi: 10.1063/5.0051423
- “Gas-filled capillary-discharge stabilization for plasma-based accelerators by means of a laser pulse”, A. Biagioni et al., Plasma Phys. Control. Fusion, vol. 63 (2021) pag. 115013, doi: 10.1088/1361-6587/ac1f68
- “Advanced Stabilization Methods of Plasma Devices for Plasma-Based Acceleration”, M. Galletti et al., Symmetry vol. 14 (2022) pag 450, doi: 10.3390/sym14030450
- “Characterisation of supersonic gas jets for different nozzle geometries for laser-plasma acceleration experiments at SPARC_LAB”. G. Costa et al., JINST vol. 17 (2022) pag. C01049, doi: 10.1088/1748-0221/17/01/C01049
- “Characterisation and optimisation of targets for plasma wakefield acceleration at SPARC_LAB”, G. Costa et al., Plasma Phys. Control. Fusion vol 64 (2022) pag. 044012, doi: 10.1088/1361-6587/ac5477
- “Shot-by-shot stability of the discharge produced plasmas in suitably shaped capillaries”., S. Arjmand et al., JINST vol. 18 (2023) pag. C04016 doi: 10.1088/1748-0221/18/04/C04016
- “Investigating of plasma diagnostics by utilizing spectroscopic measurements of Balmer emission”, S. Arjmand et al., JINST vol. 18 (2023) pag. C05007 doi: 10.1088/1748-0221/18/05/C05007
- “Spectral line shape for plasma electron density characterization in capillary tubes”, S. Arjmand et al., J. Phys.: Conf. Ser. vol. 2439 (2023) pag. 012012 doi: 10.1088/1742-6596/2439/1/012012
- “Characterization of plasma-discharge Capillaries for Plasma-based Particle Acceleration”, L. Crincoli et al., J. Phys.: Conf. Ser., vol. 2687 (2024) pag. 042006 doi: 10.1088/1742-6596/2687/4/042006
- “Effect of dielectric wakefields in a capillary discharge for plasma wakefield acceleration”, L. Verra et al., Nucl. Instrum. and Meth. in Phys. Res. Section A vol. 1072 (2025) pag. 170157, doi: 10.1016/j.nima.2024.170157
- “Femtosecond laser-induced plasma filaments for beam-driven plasma wakefield acceleration”, M. Galletti et al., Phys. Rev. E, vol. 111 (2025), pag 025202, doi: 10.1103/PhysRevE.111.025202
Contact person:
Angelo Biagioni, angelo.biagioni@lnf.infn.it, tel. (+39) 069403 2632