THz Source
THz Source
The interest in Terahertz (THz) radiation is recognized since many years for its potential to advance research in several scientific fields. In addition, THz research has many industrial prospects, so that THz activities may offer potential spin-off not only associated to condensed matter basic research, e.g., semiconductor and superconductors materials, whose characterization may have a direct impact on many technologies, but also in R&D of detectors and imaging. A great expectation for industry is the development of imaging for biomedical applications and security issues.
THz radiation lies between the photonics and the electronics bands of the electromagnetic spectrum, and it extends from 300 GHz up to 10 THz. THz is non-ionizing and highly penetrating in a large variety of dielectric materials, e.g. plastic, ceramics, paper. The THz part of the spectrum is energetically equivalent to many important physical, chemical and biological processes, including superconducting gaps, exotic electronic transitions and protein dynamical processes.
THz sources based on particle accelerators allow to rise up the average and peak power by many orders of magnitude respect to conventional sources, and extends the spectral range up to the Mid-Infrared (100 THz, MIR), making the whole spectral region to be accessible to different frequency- and time-domain experiments.
In particular, at SPARC_LAB, THz radiation is produced through radiative phenomena based on ultra-relativistic electron bunches. Currently, both Coherent Transition Radiation (CTR) and Coherent Diffraction Radiation (CDR) are generated from ultra-short (sub-ps, down to 20 fs) electron bunches as those produced and manipulated at the SPARC high brightness photo-injector, allowing the delivery of broadband pulses with femtosecond shaping, and with the possibility to store a high energy in a single pulse [1].
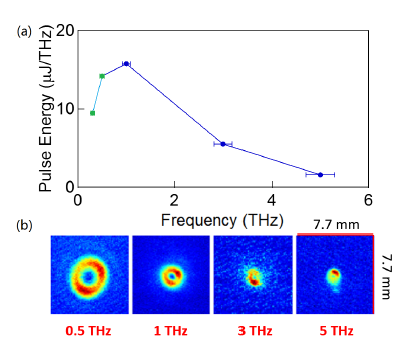
Figure1. Broadband THz generation: (Top) spectral distribution sampled by THz filters. The error bars represent the bandwidth of the bandpass filters; (Bottom) Transverse intensity distributions of CTR in the focus of the parabolic mirror for different wavelength. Since the used power meter (THz-I-BNC GENTEC-EO) is not calibrated for frequencies below 0.61 THz, the measured energy for frequencies below this value is a qualitative assessment.
A proper optical system is needed to extract the CTR and transport it down to the detection apparatus, consisting in a diamond window and a collection of aluminum coated parabolic .
The coherent THz source is currently characterized by measuring the radiation spectrum in air (or dry air environment) both through a set of discrete custom THz filters and an interferometer (either Martin-Puplett or Michelson) [5]. The dry air system contributes to reduce water absorption allowing to measure radiation from very low charge bunches, of the order of 10 pC, needed for the production of ultra-short, fs-scale, electron bunches.
In addition, taking advantage from electron beam manipulation techniques, such as velocity bunching and laser comb, high power, narrow-band radiation can be also generated [2,3].

Figure2. (Top) Multi-bunch, comb-like, electron beam with ramped charge distribution: longitudinal phase space (left) and current profile (right). Electron beam parameters for each bunch are listed in the table. (Bottom left) Interferometric pattern, typical of a multi-beamlets pulse; (bottom right) THz radiation spectrum.
Finally, high brightness electron beams also permit the possibility to extend the emission towards the MIR, having a unique source covering three decades in wavelength from 1000 microns to 1 micron. This provides a unique chance to realize THz/MIR-pump/THz/MIR probe spectroscopy, a technique practically unexplored up to now.
Beyond these user-driven applications, coherent THz radiation is also used as longitudinal electron beam diagnostics to reconstruct the beam charge distribution [4].
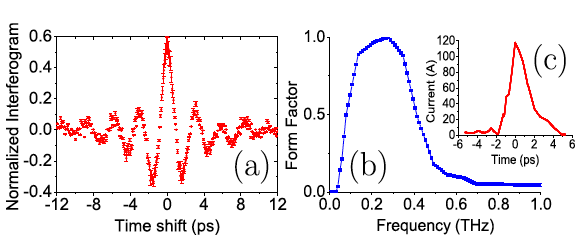
Figure3. (a) Autocorrelation function as measured through a Martin-Puplett interferometer; (b) Bunch form factor; (c) Retrieved longitudinal bunch profile by applying Kramers-Kronig technique: 1.4 (0.1) ps rms.
User experiments
User experiments
The advanced THz radiation, produced at SPARC_LAB, is characterized by few tens of fs duration pulses, few hundreds of MW peak power, several tens of micro-Joule energy per pulse, broad and narrow bandwidth. Typical THz radiation parameters achieved at SPARC_LAB are listed in Table 1.
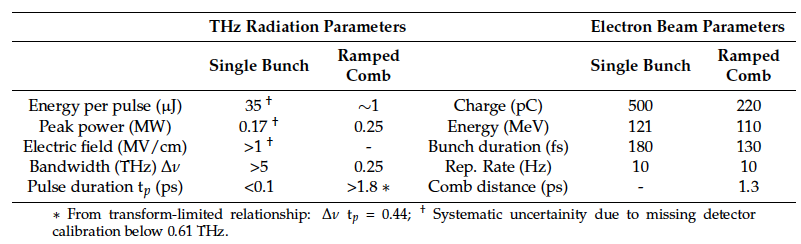
Table1. SPARC_LAB THz sources: radiation and electron beam parameters for a single bunch and a ramped five-bunches train.
Beyond the use of THz radiation for electron beam longitudinal diagnostics, all the features mentioned make the SPARC_LAB THz radiation strongly competitive with respect to conventional THz sources and extremely suitable for investigating non-linear THz spectroscopy and for testing novel detectors and materials.
First User Experiment
First User Experiment
The THz source at SPARC_LAB has the remarkable characteristic of being able to produce MV/cm electronic fields, that is, atomic electric fields. Such intense electric fields can, in fact, excite fundamental quantum statuses of the examined system, allowing non-linear optic experiments.
In particular, thanks to the SPARC_LAB THz source, the research team coordinated by Stefano Lupi, from the “Sapienza” University in Rome and Enrica Chiadroni from the INFN National Laboratory of Frascati successfully investigated the non-linear properties of topological insulators. These materials possess the important quality of acting like electric insulators inside materials, showing, at the same time, conductive status on the surface. Another property hypothesized but never investigated before concerns the optic response of non-linear topological insulators to radiation impulses in the THz frequency region. This experiment has been performed with the SPARC_LAB THz source, investigating Bi2Se3, and results have been published on the prestigious review Nature Communications [6].
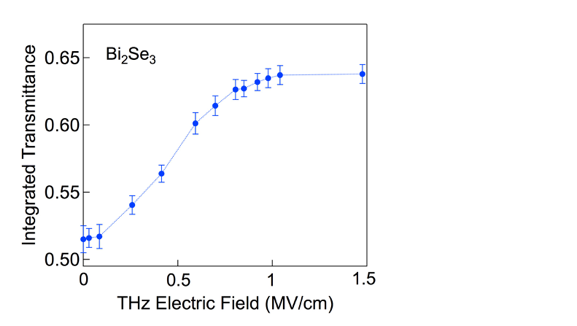
Figure4. Intensity transmitted by the Bi2Se3 topological insulator sample in the THz electric field.
Measurements taken with different THz electric fields showed the radiation intensity of the sample (a thin Bi2Se3 layer) was constant for fields up to a few tens of kV/cm, which is the linear region of response of the material. As the electric field associated to the impulse of the THz radiation grew, transmitted intensity started to grow as well, increasing of about 25% with a high electric field saturation trend. The induced transparency phenomenon in the THz spectrum region is the experimental evidence of the non-linear optic behavior of the Topological Insulator Bi2Se3.
CalipsoPlus Program
CalipsoPlus Program
Thanks to the peculiar features shown, the SPARC_LAB THz source has been inserted in the CalipsoPlus program, which provides dedicated beam time to external users.
Publication highlights:
[1] “The SPARC linear accelerator based terahertz source”, E. Chiadroni et al., Appl. Phys. Lett. vol. 102 (2013) pag. 094101, doi: 10.1063/1.4794014.
[2] “Characterization of the THz radiation source at the Frascati linear accelerator”, E. Chiadroni et al., Rev. Sci. Instrum. vol. 84 (2013) pag. 022703, doi: 10.1063/1.4790429.
[3] “Tailoring of Highly Intense THz Radiation Through High Brightness Electron Beams Longitudinal Manipulation”, F. Giorgianni et al., Appl. Sci. vol. 6 (2016) pag. 2, doi: 10.3390/app6020056.
[4] “Bunch Length Characterization at the TTF VUV-FEL”, E. Chiadroni, Ph.D. thesis TESLA-FEL 2006-09 (2006).
[5] “Characterization of the THz source at SPARC”, E. Chiadroni et al., Proc. of IPAC 2010, TUOARA03, Kyoto (2010).
[6] “Strong nonlinear terahertz response induced by Dirac surface states in Bi2Se3 topological insulator”, F. Giorgianni et al., Nature Communications vol. 7 (2016) pag. 11421, doi:10.1038/ncomms11421.
Contact person: E. Chiadroni, enrica.chiadroni@lnf.infn.it, tel. (+39) 069403-2289